Wide-field epi-illumination fluorescence microscopy is an indispensable tool in many biological studies due to its high sensitivity and high specificity (Fig. 1). Especially, single- molecule imaging is a powerful approach for quantitative analysis since it is capable of counting the number of molecules in a digital fashion and observing the dynamics of individual molecules in real-time. This can be used for super-resolution fluorescence imaging when the density of molecules is properly controlled by photoactivation.
To visualize individual fluorescent molecules, it is critical to ensure a high signal-to-noise ratio. Total internal reflection fluorescence (TIRF) microscopy has been widely used for single-molecule imaging since the late 1990s. A shallow evanescent field excites near the surface selectively, which minimizes unwanted out-of-focus fluorescent signal (Fig. 1). However, TIRF microscopy is only capable of probing the bottom most ~1– 2 μm of the cell, i.e. not suitable for imaging inside cells. Note that confocal laser scanning microscopy is not adequate for low-light applications because the high excitation power results in severe photobleaching and photodamage, and the limited number of photons from a single emitter leads to a poor signal-to-noise ratio of images.
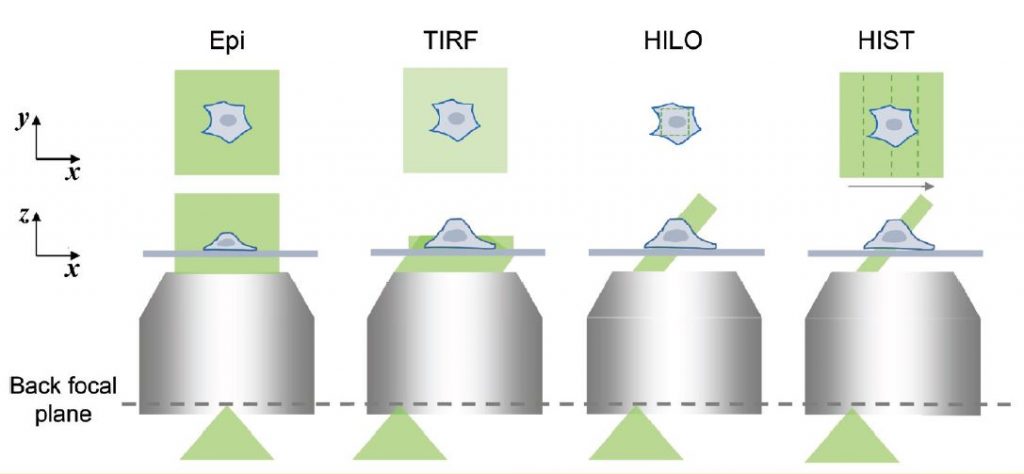
In 2008, highly inclined and laminated optical sheet (HILO) microscopy was reported for 3D single-molecule imaging. A basic idea was to use a thin illumination that was generated by an incident beam passing through the center of the imaging plane refracted at glass/water interface with an angle slightly smaller than the critical angle (Fig. 1). This inclined beam reduces out-of-focus background signal and allows high contrast 3D imaging. Because of its simplicity, HILO microscopy has been the most common imaging tool for greater imaging depth in single-molecule imaging.
However, a usable field-of-view (FOV) of HILO is less than 20 × 20 μm2, which is much smaller than the average size of human cells. This is because in HILO illumination, the beam thickness is closely related to the diameter of illumination beam, i.e., a thinner illumination always results in a smaller imaging area.
Professor Kyu Young Han and his graduate student, Jialei Tang, have overcome this limitation by sweeping a highly inclined beam elongated in one direction in conjunction with a confocal slit while maintaining advantages of HILO imaging.
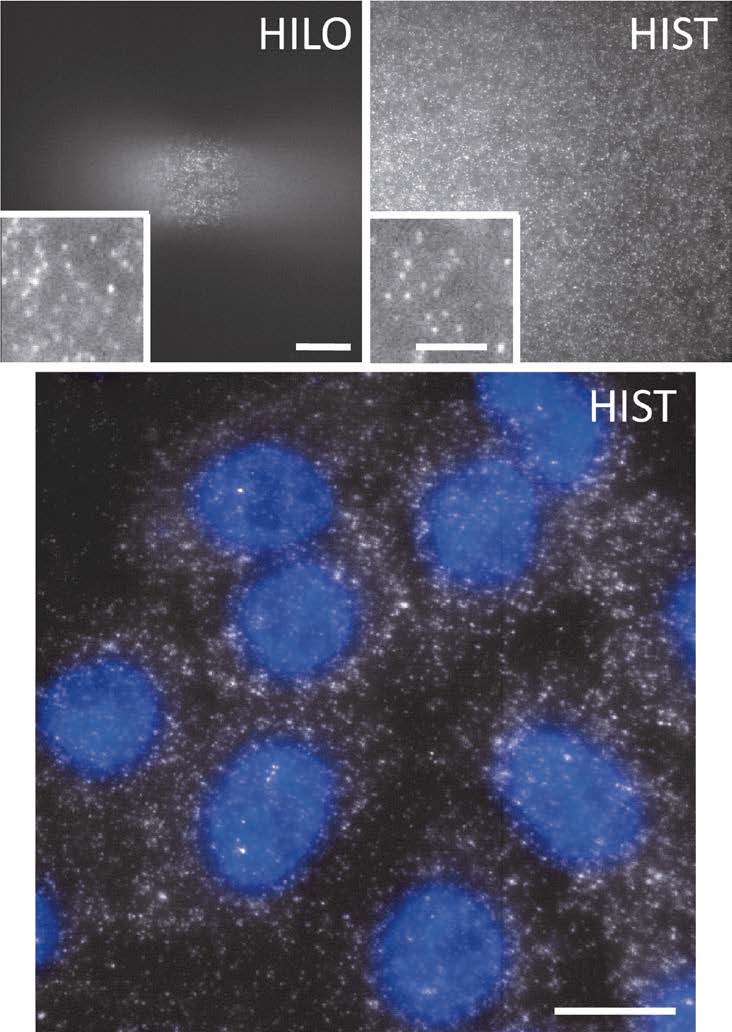
First, they created an elongated beam using a pair of cylindrical lenses and sent it to a microscope with a high incidence angle like HILO illumination. This approach generated a tile-like beam elongated along the y axis on the sample plane. Next, in order to extend the imaging area, the tile beam was swept along the x-axis by rotating a galvo mirror. Since the highly inclined beam produced out-of-focus background and blurred the image, a confocal slit is necessary, which should move along the beam sweeping. Fortunately, current state-of- art scientific grade CMOS cameras provides a dynamic slit with a rolling shutter readout, which facilitated the rejection of background in real time without additional optical or mechanical components. This new imaging more than 40 times larger than conventional HILO imaging. The signal to background ratio was also improved due to the thinner beam of HIST (Fig. 2).
The team applied this new imaging technique to detect RNA transcripts with a single probe or a few probes that has significant potentials for studying gene expression and diseases (Fig. 2). Furthermore, they demonstrated that HIST imaging enabled single-molecule imaging in thick mouse brain tissues, which has been challenging due to high background.
Unlike other approaches, HIST requires only a single high numerical aperture objective lens without an additional illuminator or reflector, and is compatible with typical sample chambers. These advantages along with large FOV and high contrast will make HIST microscopy a prominent tool in biology and medicine.
For further information, see Optica 5, 1063- 1069 (2018).
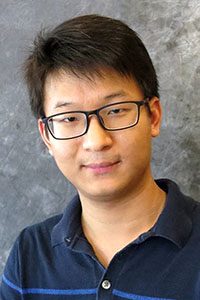