Lasers operate by stimulated emission, where a single photon is emitted per electronic transition. Two photons, with combined energy matching the transition energy, can also stimulate emission of two additional photons in a process known as two-photon gain (2PG). Just as linear (single-photon) absorption changes into stimulated emission with population inversion, two-photon absorption (2PA) changes into 2PG. The inherent nonlinearity of 2PG gives rise to interesting properties of potential two-photon lasers, such as pulse compression, self-mode locking, and unique photon statistics. Their most exciting feature arises from the fact that there is no requirement on the energies of the individual photons, so long as their sum matches the transition energy. Thus, by choosing a wide-gap semiconductor like GaN, a two-photon laser has the potential to be continuously tunable from the UV to the IR. The only requirement on the semiconductor is, like any semiconductor laser, it must have a direct bandgap.
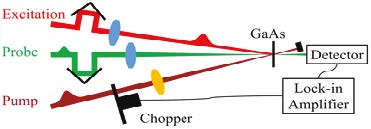
Diagram of the experimental set-up showing Pump and time-delayed Probe having very different wave- lengths. The above-gap Excitation beam is temporally positioned to create excited carriers ~10 ps prior to the arrival of the Pump.
Professors David Hagan and Eric Van Stryland, along with 2015 PhD graduate Matthew Reichert, currently a postdoc at Princeton University, have spent many years measuring 2PA in semiconductors and have determined that the already large 2PA coefficient for pairs of equal energy photons is enhanced by 2 to 3 orders of magnitude when the photon pairs have very different energies (photon energy ratios of ~10). This extremely nondegenerate (END) enhancement translates directly to 2PG, making semiconductors likely candidates for END 2PG media.
The team has already demonstrated END 2PG in optically excited GaAs via femtosecond pump-probe experiments, as shown in Fig. 1. The transmittance of a probe pulse, with a wavelengths of 977 nm, corresponding to a photon energy ~90% of the band gap, is measured versus temporal delay of an infrared pump pulse at 7.75 μm. With the above gap excitation at 780 nm turned off, 2PA loss is observed, while with the excitation on a few picoseconds before the pump, 2PG is observed.
These experiments constitute the first demonstration of highly nondegenerate 2PG in any medium. An END 2PG coefficient of more than 25X the degenerate, i.e. equal energy 2PA coefficient, was measured, confirming that nondegenerate enhancement of 2PA in semiconductors enhances 2PG as well. Such enhancement may open the way towards the development of two- photon semiconductor lasers.
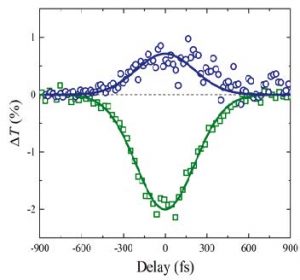
Pump-probe experiments without excitation showing 2PA (green squares), and with excitation showing 2PGain (blue circles).
Note that the 2PG observed was not a net gain, as there are several background absorption processes that combine to exceed the maximum 2PG observed so far. These losses include free- carrier absorption, which is typically high in the mid infrared, and three-photon absorption (3PA). It turns out that 3PA is also enhanced in the END case and this places an upper limit on the irradiance and thus the obtainable 2PG. In bulk semiconductors, it appears that this may preclude a 2-photon laser; however, the team’s theoretical calculations indicate that with proper design, another order of magnitude enhancement of 2PG (and 2PA) can be obtained in multiple quantum-well structures while reducing free-carrier absorption and the eliminating the enhancement of 3PA.
Van Stryland, Hagan and their students are currently investigating the 2PA and 2PG in waveguide structures of multiple quantum well structures grown at Sandia National Labs. The results of these experiments will help chart the course for the realization of semiconductor lasers with net gain.
Of course, even after overcoming the issues of parasitic loss and showing that net gain is possible, several challenges to advancing to a free-running, 2-photon semiconductor laser device remain. Most significant of these is the suppression of single-photon emission. Although this is not currently on the team’s list of priorities, long-term solutions may include selective wavelength suppression, such as by absorption or by the incorporation of photonic bandgap structures in the laser devices. Should the CREOL research team succeed in its goal of demonstrating net 2PG, it is certain that many researchers and laser manufacturers will have a strong interest in solving these practical problems.
For further information see M. Reichert, et al., Phys. Rev. Lett. 117, 073602 (2016)
Authors
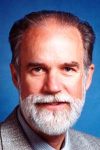
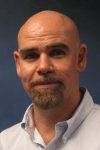
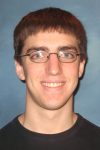