Since its invention in the late 16th Century, the optical microscope, has become an essential tool in modern biology, leading to numerous discoveries. Fluorescence is probably the most important optical readout mode because of its high sensitivity and specificity, compared with absorbance and reflectance. Whereas the remarkable breakthroughs based on fluorescence contrast, including the recent Nobel Prizes in green fluorescence protein and super-resolution microscopy, are only a couple of decades old, the fundamental optical design and data collection in the optical microscope have undergone very little change in the last 400 years. In the age of post genomics, the fundamental way we approach biological questions has been changed: more questions can and are necessary to be answered by massive data sets. In the quest of acquiring images with bigger field-of-view at a faster recording rate, microscope lenses with more complex design and sensors with higher readout speed have been developed. These approaches, reaching the performance bottleneck, make the system cost orders of magnitude higher than the common setups.
The two recent research projects in Sean Pang’s Optical Imaging System Lab have demonstrated alternative approaches by combining the signal reconstruction techniques with unique systems design to improve the performance in microscopic imaging at a fraction of the cost.
In the first project, Pang’s group have demonstrated a microscopy configuration achieving 200 frames per second with a consumer grade imager whose frame rates is 40 Hz, shown in the figure. The “UCF” logo was translated at speed of 2 mm/sec. Each letter of the logo has a dimension roughly 50 microns. If low-frame rate camera were used to capture the scene, we would record a blurred image with the fine features lost due to the motion. To restore the highframe rate from low-frame rate measurement, structured illumination was employed as a coding mechanism in the prototype. Similar to super-resolution microscopy, where structured illumination has been employed to introduce a spatial frequency shift, the temporally coded multiplexing illumination channels the high temporal frequency components into low frame rate detection.
The cost of such a system is essentially the same as a conventional epi-fluorescence microscope, and the setup requires minimal modification. Many fundamental biological processes, especially neural activities, which have a time scale of several millisecond, and could previously be imaged only by use of an EMCCD camera, can now be recorded by use of a conventional sensor. In addition,the imaging methodology can work with any cameras, including EMCCD or sCMOS. With the topof-the-line sensor, the frame rate could reach 100 mega frame per second, boosting the throughput in microfluidic analysis and opening new imaging possibilities.
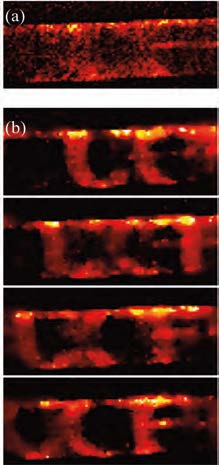
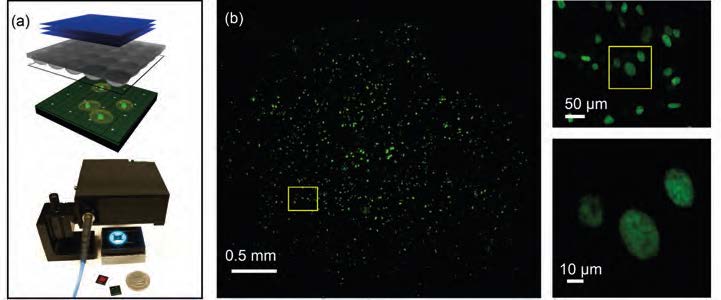
Another effort in redesigning the microscope aims to increase its field-of-view (FOV). Due to limits imposed by optical aberrations, common microscope objectives (20×) have an FOV less than 1 mm. To scale up the FOV, additional lens elements are necessary, leading to a lower transmission objective with higher design and material cost. Pang’s group came up with a compact microscopic imaging system utilizing the Talbot effect to project a grid of excitation light spots focused onto the sample which is placed on a CMOS sensor chip (see Figure 2). The fluorescence emission associated with each focal spot is collected by the sensor chip and are used to form a sparsely sampled fluorescence image. By scanning the Talbot focal spot grid across the sample and collecting a sequence of local images, a high-resolution fluorescence image can be reconstructed. In contrast to a conventional microscope, the collection efficiency, resolution, and FOV of the system are not tied to each other for this technique. As a result, the FOV of the system is directly scalable. A resolution of 1.2 μm was demonstrated in a prototype, and the collection efficiency is equivalent to that of a conventional microscope objective with a 0.70 N.A. The FOV is 100 times greater than a 20×/0.40 NA conventional microscope objective.
As the cellphone industry drove the cost of a CMOS sensor down to ~$2 apiece, a lensless microscope will bring the system’s cost to less than $100. Due to its large FOV, high collection efficiency, compactness, and its potential for integration with other on-chip devices, FTM is suitable for diverse applications, such as point-of-care diagnostics, large-scale screening, and long-term automated imaging.
Author
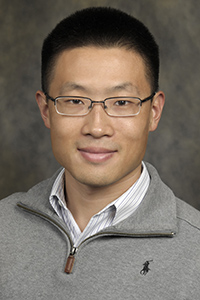
Pang joined CREOL in 2015. He leads the Optical Imaging System Lab (OISL). Pang obtained his PhD degree in Electrical Engineering from California Institute of Technology in 2013, and conducted his postdoctoral research at Duke University.